The Mechanism of Action of Azithromycin in Fighting Infections
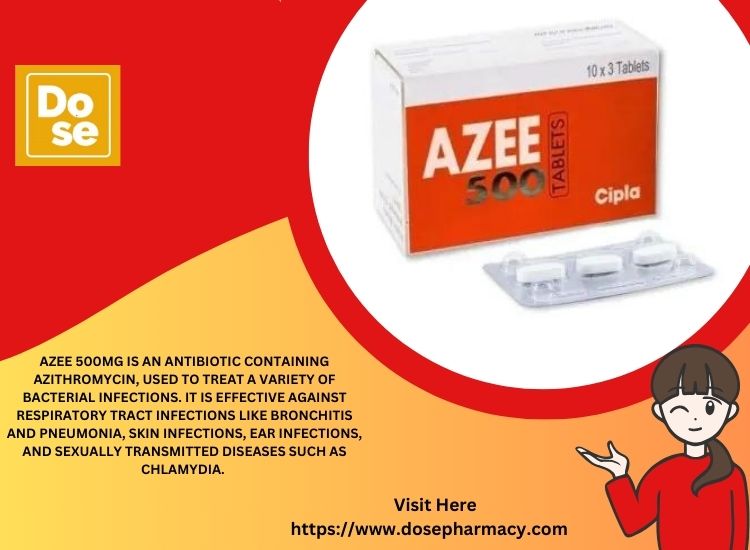
Azithromycin is a widely prescribed antibiotic belonging to the macrolide class, used to treat a broad range of bacterial infections. Known for its relatively long half-life and ability to be administered in short courses, azithromycin is a preferred option for several types of infections. Its mechanism of action is central to its efficacy in eradicating pathogens, and understanding how it works is key to appreciating its therapeutic applications and challenges. This article explores the molecular and cellular mechanisms by which azithromycin combats bacterial infections.
Overview of Azithromycin
Buy azithromycin online is a semi-synthetic derivative of erythromycin, first introduced in the late 1980s. It is commonly prescribed for respiratory tract infections, such as pneumonia, bronchitis, and sinusitis, as well as for sexually transmitted infections (STIs), such as chlamydia and gonorrhea. It has also been shown to be effective in treating skin infections, ear infections, and certain gastrointestinal infections. The drug's broad-spectrum activity makes it a versatile treatment option for various bacterial pathogens, including Streptococcus pneumoniae, Haemophilus influenzae, Chlamydia trachomatis, and Mycoplasma pneumoniae.
Mechanism of Action: Inhibition of Protein Synthesis
The primary mechanism by which azithromycin exerts its bacteriostatic effects is through the inhibition of bacterial protein synthesis. This is achieved through its interaction with the bacterial ribosome, a complex molecular machine responsible for translating messenger RNA (mRNA) into functional proteins. Azithromycin, like other macrolides, binds specifically to the 50S ribosomal subunit, a key component of the bacterial ribosome.
a. Binding to the 50S Ribosomal Subunit
Azithromycin binds to the 23S ribosomal RNA (rRNA) of the 50S subunit in bacteria. The binding site for azithromycin is located in the peptidyl transferase center of the ribosome, where the elongation of the polypeptide chain occurs. This binding interferes with the ribosome’s ability to move along the mRNA, a process called translocation, which is essential for protein elongation during translation. Without this movement, the ribosome cannot progress to add amino acids to the growing protein chain.
b. Prevention of Protein Elongation
The inhibition of translocation prevents the synthesis of essential proteins required for bacterial growth and survival. Because bacteria are highly dependent on protein synthesis for maintaining cellular functions, azithromycin effectively halts their ability to multiply. As a result, the bacteria are unable to produce critical enzymes, structural components, and toxins needed to sustain their cellular integrity and ability to infect the host. This action is bacteriostatic in nature, meaning it does not kill the bacteria directly but rather prevents their growth, allowing the immune system to clear the infection.
Bacteriostatic vs. Bactericidal Effect
Azithromycin is generally considered a bacteriostatic antibiotic because it inhibits bacterial growth rather than killing bacteria outright. However, at higher concentrations, azithromycin can have bactericidal effects against certain organisms, such as Streptococcus pneumoniae. This dose-dependent nature of azithromycin’s activity highlights its versatile therapeutic potential. In the case of susceptible pathogens, azithromycin’s bacteriostatic effect allows the body’s immune defenses, particularly phagocytic cells like neutrophils and macrophages, to clear the infection effectively.
Intracellular Penetration and Tissue Distribution
One of the unique properties of azithromycin is its ability to penetrate host cells, which enhances its effectiveness against intracellular pathogens. Unlike many antibiotics, azithromycin can enter host cells and concentrate within the intracellular compartments, including macrophages and neutrophils. This ability is particularly beneficial in treating infections caused by intracellular pathogens such as Chlamydia trachomatis, Legionella pneumophila, and Mycobacterium avium complex.
a. Phagocytic Cells and Azithromycin
Phagocytic cells, such as macrophages, are responsible for engulfing and killing pathogens. Azithromycin’s high intracellular concentrations within these cells allow it to exert its antimicrobial effects directly within the infected cells, where many pathogens reside and replicate. After being engulfed by phagocytes, bacteria are often contained within phagosomes, which can fuse with lysosomes to form phagolysosomes, where pathogens are typically destroyed. Azithromycin’s intracellular concentration is thought to contribute significantly to its efficacy in treating infections caused by intracellular bacteria that evade traditional extracellular antibiotic therapy.
b. Tissue Penetration
Azithromycin also has an extensive tissue distribution profile. It achieves high concentrations in tissues such as the lungs, tonsils, and prostate, making it particularly effective for treating respiratory and genital tract infections. In some tissues, azithromycin concentrations are several times higher than those found in plasma, which contributes to its effectiveness in localized infections.
Pharmacokinetics: Long Half-Life and Dosing Convenience
Azithromycin’s long half-life is another key factor in its clinical utility. The drug’s half-life is around 68 hours, which is much longer than other antibiotics, such as erythromycin. This prolonged half-life allows for once-daily dosing, which improves patient compliance, especially in treating chronic or recurring infections. After a single dose, azithromycin remains in the system at therapeutic levels for several days, providing continued action against bacterial pathogens.
The ability to be administered over a short course (typically 3 to 5 days) is also a significant advantage, reducing the risk of adverse effects associated with prolonged antibiotic use. This makes azithromycin a more convenient and tolerable option for patients, particularly for those who may have difficulty adhering to longer antibiotic regimens.
Resistance to Azithromycin: Mechanisms and Implications
Despite its widespread use, azithromycin is not without the challenge of antibiotic resistance. Over time, certain bacteria have developed mechanisms to resist its action, which can significantly reduce its effectiveness. The main mechanisms of azithromycin resistance include:
a. Target Modification
One of the primary mechanisms of resistance is the modification of the ribosomal target. Bacteria can alter the binding site on the 50S ribosomal subunit, preventing azithromycin from effectively binding to the ribosome. This is often mediated by methylation of the 23S rRNA, which reduces the drug’s ability to bind and inhibit protein synthesis.
b. Efflux Pumps
Efflux pumps are transport proteins located in the bacterial cell membrane that actively expel antibiotics from the cell before they can reach effective concentrations. Bacteria with upregulated efflux pumps can pump azithromycin out of the cell, preventing it from exerting its antimicrobial effects. This resistance mechanism is particularly prevalent in Gram-negative bacteria.
c. Enzymatic Inactivation
Though less common, some bacteria have developed enzymes capable of breaking down or inactivating macrolides like azithromycin. This enzymatic resistance is usually seen in some Gram-positive pathogens, including Streptococcus pneumoniae and Staphylococcus aureus.
Resistance to azithromycin poses significant challenges in treating infections, particularly in high-risk populations and regions with high rates of antibiotic misuse. Continued surveillance and prudent antibiotic stewardship are essential to combat resistance and preserve the efficacy of azithromycin.
Clinical Applications and Therapeutic Use
Azithromycin is indicated for a variety of bacterial infections, thanks to its broad-spectrum activity and favorable pharmacokinetics. Its most common uses include:
-
Respiratory Infections: Azithromycin is effective in treating conditions such as community-acquired pneumonia, bronchitis, and sinusitis, often caused by Streptococcus pneumoniae, Haemophilus influenzae, and Mycoplasma pneumoniae.
-
Sexually Transmitted Infections: Azithromycin is a first-line treatment for infections like chlamydia and gonorrhea, where it offers a convenient single-dose regimen.
-
Skin and Soft Tissue Infections: Azithromycin is effective against Staphylococcus aureus and Streptococcus pyogenes, commonly involved in skin infections.
-
Gastrointestinal Infections: The drug is also used to treat certain bacterial gastroenteritis, including those caused by Campylobacter jejuni and Shigella species.
Conclusion
Azithromycin’s mechanism of action involves the inhibition of bacterial protein synthesis through binding to the 50S ribosomal subunit, which halts bacterial growth. Its ability to penetrate host cells and achieve high tissue concentrations contributes to its effectiveness, especially against intracellular pathogens. Azithromycin’s long half-life, favorable pharmacokinetics, and broad-spectrum activity make it an indispensable tool in modern medicine. However, the growing problem of antibiotic resistance underscores the importance of judicious use and ongoing research to preserve the effectiveness of azithromycin in treating bacterial infections.